Ethan Yackulic is currently a data scientist for the USGS in Flagstaff, AZ. He recently completed his master’s degree at Northern Arizona University with Dr. Nicholas McKay in environmental science
Introduction/background
The San Juan Mountains of southern Colorado are the headwaters of the Rio Grande and San Juan rivers, massive watersheds that sustain numerous ecosystems and human communities on both sides of the continental divide, from Colorado to Mexico. An understanding of past climatic changes and associated environmental impacts is critical to developing management strategies in a region that is projected to face increasing risks of extensive tree death, forest fires, and drought, as well as diminishing crop yields, snowpack, and summer streamflow.
As human population centers continue to expand in this region (13.8% population growth in the Southwest according to the 2010 US Census), it is important to assess the potential risks associated with dwindling water reservoirs, especially with consideration of anthropogenic climate change. It is difficult, however, to understand the relationship between rising temperatures and long- term moisture availability because historical records are not long enough to account for a wide range of climatic variability. Paleoclimatic records are necessary to understand regional variability throughout the Holocene, allowing an evaluation of a variety of temperature and moisture conditions than exceed the instrumental period.
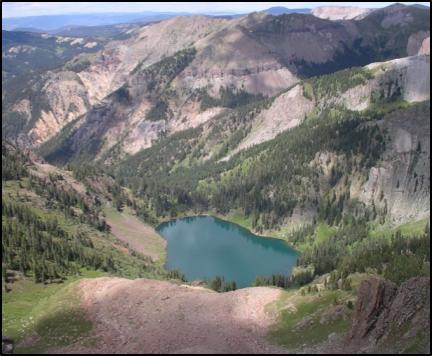
Crater Lake from up high on a calmer day. Note the moraine feature in the foreground, an artifact of the past glacial period.
To address this scientific need, I collected a suite of lake sediment cores from Crater Lake, a small alpine lake in southern Colorado. These cores represent sediments deposited between 16,000 years ago and the present and act as a proxy for historical climatic records. These data help inform our understanding of the relationship between temperature and moisture in this part of the world that is heavily dependent on snowpack availability.
Details
The first step to conducting this study was retrieving the sediment cores from the bottom of Crater Lake. A team of pack animals accompanied us on the four-mile trek, carrying all the heavy gear that we would eventually turn into a coring platform. Several people aboard the platform would take turns lifting and dropping a 25-lb hammer that slowly pushed our five-meter core tube into the lake sediment surface. When the core tube stopped moving (either because the core was full or because we hit a large object, including but not limited to: a package of glacial till, bedrock, or dinosaur bones (okay maybe not that one)), the core tube was pulled out of the lake bottom and capped at the water surface by a third member in a pack raft.
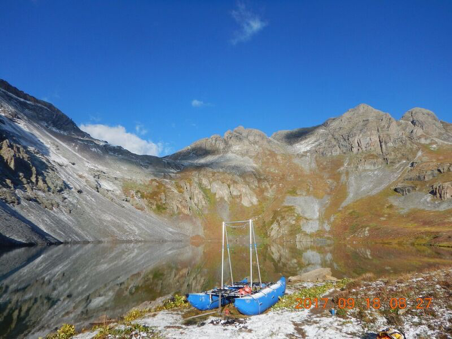
The coring platform fits up to the 3 people on board at all times and is equipped with 0 additional vessels in case of emergency.
This process often disturbed the sediment surface because of the size and weight of the core tube. However, an untampered sediment surface is incredibly important because it gives you a starting age to work backwards from when you are trying to build a chronology. Thus, surface sediment cores are often taken to fill-in the missing gap at the top of the longer cores. These cores were often taken by just one person in an inflatable raft, and Charles Mogen and I spent several summers backpacking throughout southern Colorado collecting exploratory surface cores.
Working in the lab did not carry the same luster as alpine hiking, but it did provide me an opportunity to work with a brand new hyperspectral camera that, for a short time, was the only make and model of its kind in the world. This camera measures the reflectance of light at hundreds of different wavelengths between 400-1000 nm (visible to near-infrared range). This device enabled me to infer concentrations of different photopigments (Wikipedia!) based on an established knowledge of the wavelengths at which those photopigments displayed diagnostic reflectance. For instance, chlorophyll a is known to have a reflectance minima at 660 nm. By calculating the depth of this reflectance minima relative to a flat line, I could infer the relative concentration of chlorophyll a (for more information, check these papers: Rein&Sirocko2002, Butz et al. 2015). In my study, I looked primarily at chlorophyll a and phycocyanin, a previously unresearched photopigment in paleoenvironmental studies that is only found in cyanobacteria. This methodology was relatively quick and cheap, allowing me to spend more time in the field and spend more money on other objectives (like going to Switzerland and figuring out how to use the camera in the first place!).
Results and Conclusions
The results of my study showed that spectrally-inferred concentrations of phyocyanin in the sediment cores from Crater Lake aligned with established global temperature reconstructions for the past 12 thousand years (ka). Essentially, the concentration of cyanobacteria was responding to changes in temperature: warmer periods caused a proportional increase in the amount of cyanobacteria while colder periods caused a proportional decrease. Crater Lake warmed rapidly after the end of the last ice age until around 7-8 ka, a period known as the Middle Holocene Climatic Optimum, when the climate was likely much drier and warmer than today. Temperatures remained high until around 5 ka, and there is significant evidence that Crater Lake was hydrologically closed during this time period, suggesting that the water level was much lower than at present. This is likely due to a decrease in snowpack, as winter precipitation is thought to have decreased during this period and summer temperatures were regularly warmer.
The temperature record from Crater Lake decreases after 5 ka before suddenly spiking towards the present, beginning around 1850 CE (Common Era). In fact, the temperature increase between 1913-2013 CE was greater than any other hundred-year period since the end of the last glacial period! This informs my strongest takeaway from my work at Crater Lake; the rate of change over the modern period is only comparable to the greatest period of climatic instability during the entire Holocene epoch. This period is uniformly recorded as a period of enhanced fire frequency, as vegetation regimes were replaced rapidly to keep up with the changing climate. Furthermore, modern temperatures are quickly approaching the highest recorded values during the entire Holocene at Crater Lake, suggesting that the recorded loss of snowpack in the Rocky Mountains and the heightened risk of forest fires over the past decades may be a sign of the new normal, and drier conditions may be here to stay.
This work is licensed under a Creative Commons Attribution-NonCommercial-ShareAlike 4.0 International License.